Magnetic resonance imaging (MRI) scanners, which examine soft tissue in the body using strong magnets and radio frequencies, were first used in the 1970s. Since then, MRI technology has mushroomed; the machines are available in many hospitals, and some 10 million people a year get an MRI scan, according to the International Society for Magnetic Resonance in Medicine. It is a safe, non-invasive way for doctors to peer beyond the skin, especially useful for diagnosing cancers or brain and cardiovascular problems.

Images of a rat brain. The animal is being examined in the 900 MHz magnet in the background.
Developed from the more established field of nuclear magnetic resonance (NMR), MRI is still a young technology. The National High Magnetic Field Laboratory is at the forefront of MRI technique development. In fact, the lab's 900 MHz NMR magnet is the strongest MRI scanner in the world. Nobody reading this article, however, would qualify as a patient: The machine accommodates only the most petite patients – mice, birds, hamsters and rats.
If you think that's a drawback, think again: What the machine lacks in space, it makes up for in power. Scientists measure magnetic field in units called tesla. A fridge magnet, for example, generates a field of about 0.01 tesla. A typical hospital MRI magnet is about 1.5 tesla. Our 900 MHz magnet is much, much stronger: 21.1 Tesla. To make a stronger MRI magnet, you narrow the width of the bore – the middle of the magnet, where the patient goes. That's why the bore of our super magnet is so narrow (105 mm wide, or about 4 inches) compared with much weaker hospital MRIs.
Humans may not fit into this MRI machine, but they will surely benefit from it. Scientists use it to study a wide array of human diseases and disorders, from Parkinson's to cancer, from muscle degeneration to Lou Gehrig's disease, from brain injuries to drug abuse. Standing in for human patients are animal models, ranging in size from fruit flies to rats. In this article you can read about some of the research done is this amazing instrument, and how people stand to gain from the work done by these scientists and their furry, feathered or even antennaed patients.
Feathered Friends Help Scientist Learn about Tobacco
True or false: Nicotine is bad for you.
No matter how you answered, you're correct. Nicotine is good for you. And bad for you. It all depends, at least in part, on the age at which you are exposed to nicotine.

Susanne Cappendijk and zebra finches.
It's a medical mystery that FSU College of Medicine Assistant Professor Susanne Cappendijk is trying to unravel with the help of the MagLab's 900 MHz magnet and some fine, feathered friends.
Cappendijk has been researching the long-term effects of drugs such as nicotine on the brains of zebra finches. Why zebra finches? Turns out these beautiful, bitty birds, weighing in at around 15 grams, have to learn to sing in a way not unlike how babies learn to talk. The areas of their brains involved in song learning and memorization (cognitive functioning) are similar to comparable structures in humans. Also, these animals mature relatively quickly: Within 90 days after hatching they are sexually mature. So, long-term studies in finches can be performed in a shorter time span than studies with rodent and primate models.
In pregnant women, nicotine readily crosses the placenta and induces growth and neurobehavioral abnormalities (disturbed cognitive functioning) in their children. Those deficits persist at least up to 1 year of age, according to some studies, while other studies suggest they may continue into late childhood or adolescence.
In contrast, research has shown that nicotine can actually enhance memory and other neurological processes in patients suffering from neurodegenerative disorders such as Alzheimer's disease.
Cappendijk's finches are helping her figure out why young and old brains respond so differently.

One of Cappendijk's finches.
"What we are doing in my lab is trying to search for the underlying mechanisms of actions in the developing zebra finch brain," she says. "Why is [nicotine] so damaging when you are young and developing your brain, while when you are, for example, an Alzheimer's patient, it's beneficial to your brain?"
Working with the MagLab's Sam Grant, an assistant professor of engineering at the FAMU-FSU College of Engineering, Cappendijk examines finches before and after injecting them with the drug. She monitors various changes, including behavior, movement, song patterns, eating and weight. She also looks at what's going on in the brain: That's where the MagLab's "900" comes in, the most powerful MRI machine in the world.
In the 900, Cappendijk scans the finches' brains before injection, and then brings them back a few weeks later. "We rescan the animal and then we have two templates we can put on top of the other, and we can see how nicotine can affect brain nuclei," she explains.
"I can see that a volumetric change in some brain areas occurs using the 900, but I can't see what the change is due to," Cappendijk says. To answer that "why" question, the animal must be sacrificed and its brain examined. Cappendijk and her students look for genetic changes and for the neural pathways nicotine is acting on, which potentially differ for young and old finches.

Brain slices from a zebra finch.
For a magnet of its strength, the 900 has a very large experimental space – plenty big to fit live finches. This not only allows Cappendijk to design better experiments, it also decreases the number of birds she needs to sacrifice.
"Before I had the option of going to the 900, I had to sacrifice animals before [injection] to make a baseline, and I had to sacrifice animals after," Cappendijk explains. "But the problem is that an animal is not its own control, so there could be an inborn genetic difference present, which might affect the data interpretation. Using the 900 allows us to use an animal as its own control, which is very important."
Cappendijk says these studies, funded by the James and Esther King Biomedical Research Program, the First Year Assistant Professor Grant Award and FSU's Department of Biomedical Sciences, will lay the foundation for her long-term research goal: contributing to the understanding of the role of the nicotinic receptors in age-related neurodegenerative disorders and examining how nicotinic therapies can treat these disorders, without the toxic side effects of nicotine.
Jeong-Su Kim, an assistant professor in FSU's College of Human Sciences, studies sarcopenia, the atrophying of skeletal muscle generally brought on by aging. For his studies, Kim uses older rats and mice whose muscles have begun to experience the metabolic and microstructural changes that occur as animals age.
From in vitro to in vivo: New Frontiers for Studying Muscle Degeneration

Kim (facing camera) and his postdocs perform surgery on an animal.
Kim is collaborating with MagLab engineer Sam Grant to develop new techniques for researching this condition, which until now has been studied almost exclusively in vitro, i.e., removing tissue from human or animal patients, in order to analyze the samples at the cellular level. In this way scientists do crossover studies that compare a group of younger animals to a group of older animals.
But in vitro crossover studies have their limits. They're not practical for studying the progression of muscle deterioration over months or years.
"Pretty much all the cell-level sarcopenia study is done by in vitro tissue," said Kim. "We want to do in vivo muscle fiber size determination using magnetic resonance, especially in the 900 MHz magnet. The 900 is sensitive enough to actually estimate change in muscle cell size without sacrificing the animal, so we can do longitudinal studies with the same animal over the years – maybe from when it's born to when it dies – seeing what's happening in the cell size."

MRI image of the skeletal muscles of a rat's hind leg.
In sarcopenia, the skeletal muscle cells shrink, causing loss of muscle mass, strength and functionality. Diet and exercise can slow this process and improve quality of life for seniors; Kim is studying proteins and genes involved in skeletal muscle regeneration to see how exercise can reduce the rate of sarcopenia.
Kim doesn't have any answers yet; they are still developing and testing techniques for exploiting this very powerful magnet. But in vivo experiments promise to provide new insights into sarcopenia and open up entire new areas of research.
"I was so excited at the beginning when I heard what's possible [with the 900]," said Kim. "It's going to be a slow process, but I think it's doable."
Seeking Alternatives to Chemotherapy
MagLab biophysicist Victor Schepkin uses the 900 MHz NMR magnet to study brain tumors. In research funded by the National Institutes of Health, Schepkin is doing two kinds of MRI: diffusion MRI and sodium MRI.

Victor Schepkin prepares to insert a probe containing a rat into the 900 MHz magnet.
In general, MRI machines take pictures of water. The magnet is tuned to find the hydrogen protons, so it can make a map of all the H2O in your body; that's why it's so good at taking pictures of soft tissue, which is full of water, rather than your skeleton, which is (forgive the pun) bone dry.
By contrast, diffusion MRI tracks not just the location of water in the body, but the movement of that water. Schepkin uses this technique to study the effects of chemotherapy in rats with brain tumors. The images below depict water diffusion in the brain of one such rodent. The scan on the left shows the brain prior to chemotherapy; the lighter region is the tumor. The image on the right shows the same part of the brain four days after chemotherapy. The lighter shading in the tumor on the right indicates more water is flowing around those tumor cells, a sign they are breaking down and that the treatment is working.
"This is the very first sign that the treatment is working and that the tumor will shrink in the near future," says Schepkin.
Hydrogen protons aren't the only things MRIs can identify. Among a few other elements, they can also pinpoint sodium, essential to a number of biological functions. In fact, Schepkin has made some exciting images doing sodium MRI on rats with brain tumors.
Diffusion MRI is currently in clinical trial and will soon be used widely in hospitals, but the power of sodium MRI remains unknown for now, Schepkin says.
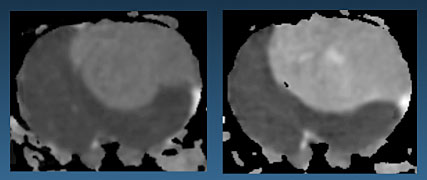
Images of a rat brain made with the 900 MHz NMR magnet. The image on the left shows the brain prior to chemotherapy; the lighter region is the tumor. The image on the right shows the area four days after chemotherapy; the lighter shading indicates more water is flowing in those tumor cells, a sign they are breaking down and that the treatment is working.
Chemotherapy, of course, triggers death in cancer cells. Unfortunately, it also destroys some perfectly good tissue. But Schepkin's research suggests there may be a better way of killing cancer cells. His scans indicate that after chemotherapy takes place, but before the cells break down, the sodium balance in those cells is destroyed. Only afterwards do the cancer cells begin to die.
"This is a very exciting finding because of the consequences it could bring," says Schepkin. "It suggests that our goal in cancer therapy should be different."
In other words, there may be an alternative to chemotherapy, which essentially requires injecting poison into the body. A more targeted approach might be to somehow induce a sodium imbalance in those cells instead, precisely targeting the cancer while sparing healthy cells from the blunt instrument of chemotherapy.
"It means we can destroy cancer cells by a different way than we did before," said Schepkin. "We're learning some clues about a new mechanism."
Better Diagnoses for Brain Disorders
Neurodegenerative diseases such as Alzheimer's and Parkinson's are not only devastating to victims and their families, but also baffling to scientists and doctors. Medical professionals don't yet fully understand what causes these diseases or what happens in the brain when they strike. They are also notoriously difficult to diagnose; doctors rely largely on patient behavior to make their determinations because there is no way to identify the diseases with certainty. Although positron emission tomography (PET) scans can help, the information those scans yield is limited: They reveal which areas of the brain are functional, but can't provide structural information and lack high resolution.

MRI scan of the hippocampus of an Alzheimer's patient. Scale on right measures 1.5 cm.
Researchers at the Mayo Clinic and the MagLab hope to help change that.
In the first collaboration of its kind, the MagLab's Sam Grant and Florida State University have teamed up with the Mayo Clinic in Jacksonville, Florida to see how effective MRI scans can be in detecting these disorders.
The clinic and Drs. Katherine Schweitzer, Dennis Dickson and Zbigniew Wszolek have provided dozens of samples of brain tissue from deceased Alzheimer's and Parkinson's patients who have donated their bodies to science. Grant and his colleagues are making highly detailed MRI scans of these samples in the powerful 900 MHz ultra-wide-bore magnet, hoping to find biomarkers that doctors can one day use to diagnose living patients.
In the samples from Alzheimer's patients, the researchers are zeroing in on the hippocampus, a curved structure located deep in the brain's temporal lobe that is the center for memory and learning. Long before patients exhibit any behavioral symptoms, Alzheimer's disease attacks the hippocampus, destroying nerve cells and the synapses between them. By the time a patient starts to have noticeable symptoms (memory loss, confusion, mood swings), tissue destruction has spread to other areas of the brain.
The disease leaves traces in the form of protein deposits. Beta-amyloid plaque builds up between neurons, clogging neural pathways. That "gunk" is one of the features that the researchers hope to identify on the MRI scans.
Grant, an assistant professor at the FAMU-FSU College of Engineering who performs MRI work at the lab, is hoping the 900 MHz magnet will be able to identify deposits of metals such as iron, copper and zinc that tend to get stuck in the plaque. Also, the MRI scans should reveal the shrinkage that a diseased brain undergoes as the cells die off.
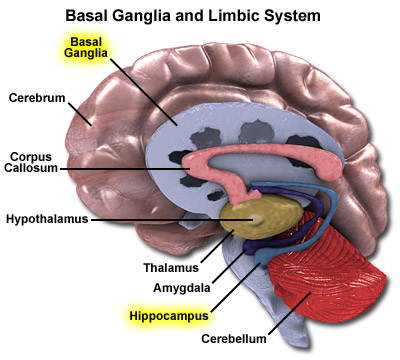
Grant hopes MRIs will reveal signs of Alzheimer's in the hippocampus and signs of Parkinson's in the basal ganglia of the human brain.
"I'm hoping that the strength of the 900 magnet can be leveraged to help diagnose these diseases in the future," said Grant, "and I'm excited about the prospect of translating this research into a technique that physicians can use with patients."
For Parkinson's patients, the researchers hone in small structures in the basal ganglia, a group of nuclei at the base of the forebrain. One of these structures, the substantia nigra, produces dopamine, a neurotransmitter that controls movement and balance. When Parkinson's attacks this structure, dopamine levels in the brain drop, eventually resulting in the telltale Parkinson's symptoms – stiffness, tremors, difficulty moving.

MRI scan of the substantia nigra of a Parkinson's patient. Scale on right measures 1.5 cm.
Previous studies have shown heavy deposits of proteins and iron in the Parkinsonian brain. The 900 scanner, powered by a massive 21.1-tesla magnet, should pinpoint those areas where metal accumulation has impacted neurons, Grant says.
After making MRI scans of the Mayo Clinic brain samples, pathologists from the clinic will do histological studies on the tissue to see if they find on a cellular level what the researchers suspect they can identify on a structural level.
If the findings are confirmed, much work still lies ahead. After all, the goal is not diagnosing the dead, but the living. One day, perhaps, doctors will be able to identify and track these diseases with high-resolution MRI scanners, possibly identifying them far earlier in their progression than is now possible.
"We're hoping to prove that you can do this work in vivo as well – if you have the resolution in order to do it," said Grant.
This article has discussed only a few of the research projects in the world's largest MRI scanner. You can take a look at some of the other research being done in the instrument in our list of some of the other MRI research projects at the MagLab.
Thanks to the Magnet Academy's scientific adviser on this article Sam Grant, an assistant professor of engineering with the Florida A&M University/Florida State University College of Engineering and an expert in MRI at the Magnet Lab.
By Kristen Coyne