Many of us believe our daily experience with magnets begins and ends on the refrigerator door. There's that cute shot of the kids in the magnetized frame, the clip holding the grocery list, the realtor's business card and the haiku composed of magnetized words.
In fact, those are just one of several types of magnets that span a broad range of sizes, shapes, materials, strengths and applications. You may not see them, but magnets are everywhere in our car motors, phones, tape recorders, credit cards, stereo speakers and computers, to name just a few practical applications. That's not even to mention the enormous and enormously powerful magnets used at the Magnet Lab and other research institutions.

Physics Factoid
Scientists believe the Earth's magnetic field is a result of convection currents in the planet's liquid outer core, which makes a good conductor because it is made up largely of iron. This is called a geodynamo.
Some magnets last lifetimes, others come and go in the blink of an eye. Some are multi-ton behemoths, others only visible through a microscope. Some are molded by nature, others by man. Magnets also span a vast range of possible magnetic field strengths.
On the following pages we'll review the basic types of magnets. But remember that there are properties that all magnets have in common. They all exert a magnetic force on each other. They all have a south pole and a north pole (just like the Earth, which itself is a magnet); opposite poles attract, like poles repel. By convention, magnetic field lines point away from a north pole of the magnet and towards the south pole. These fields are what produce forces on other magnets that follow specific physical laws.
Permanent Magnets: A Study in Sticktoitiveness
A lodestone attracting iron filings and nails.
There are two basic kinds of magnets permanent and temporary. We'll take on permanent first which is appropriate, because before humans could invent and exploit temporary magnets, they had to discover the permanent kind first.
People first happened upon magnets sometime before 600 BC with the discovery of the mineral magnetite, a naturally occurring magnet. One type of magnetite, lodestone, has the additional property of polarity it aligns itself with the Earth's magnetic field. The lodestone made possible the invention of the compass, which was used in navigation as early as 1086.
Unlike temporary magnets, the permanent ones (such as those on your fridge) stick around for awhile (no pun intended). While they may not last forever, you often have to go to some effort to demagnetize them. You can't turn them on and off with the power switch. Permanent magnets all belong to a class of materials referred to as ferromagnetic.
The Earth is a magnet.
The other major difference between permanent and temporary magnets is what the magnetic fields look like on an atomic level. These are two different phenomena entirely.
All magnetism comes down to electrons. In the electromagnets we'll discuss in a minute, magnetic fields result from electron flow through a conductor. In the case of permanent magnets, it's the spinning of the electrons that creates magnetism, not their movement through a conducting material.
You know that the Earth is a great big magnet. Well, electrons are teeny tiny ones. They have a north and a south pole, too, and spin around an axis. This spinning results in a very tiny but extremely significant magnetic field. Every electron has one of two possible orientations for its axis.
Most materials are either naturally magnetized, or can be induced to be magnetic. In most materials, atoms are arranged in such a way that the magnetic orientation of one electron cancels out the orientation of another.
Iron and other ferromagnetic substances, though, are different. Their atomic makeup is such that smaller groups of atoms band together into areas called domains, in which all the electrons have the same magnetic orientation. Inside each of these domains, the electrons are oriented in the same direction. But the iron is not magnetic because these domains themselves are not aligned.
Magnetic domains
If you put that piece of iron within a magnetic field, though, the domains all begin to point in the same direction. The result? A magnet! A permanent magnet is nothing more than such an object in which all the domains are aligned in the same direction. There are only four elements in the world that are ferromagnetic at room temperature and can become permanently magnetized: iron, nickel, cobalt and gadolinium. (A fifth element, dysprosium, becomes ferromagnetic at low temperatures.)
Open our interactive tutorial and try this yourself!
Your new magnet could remain magnetized for a good while, depending on what it is made of. Pure iron, for example, will stay magnetized just a few minutes, although magnetization of many iron alloys will be much more "permanent."Ceramic and alnico a combination of aluminum, nickel and cobalt are two common types of permanent magnets. But even more permanent magnets could lose their magnetism with, say, a good swift blow from a hammer to knock the aligned domains out of alignment. When it comes to magnets, "permanent" doesn't necessarily mean "forever"!
Temporary Magnets: When is a Magnet Not a Magnet?
Physics Factoid
Naturally occurring magnets were formed when lightning struck magnetite on Earth.
Though permanent magnets can last a long time, they fall short in the power department. Scientists measure magnetic strength in units called tesla and gauss; one tesla equals 10,000 gauss. Your fridge magnet is a modest 10 gauss. The Earth's magnetic field is about .5 gauss (or 0.00005 tesla). The most powerful permanent magnets available produce fields about 1.5 tesla. While that's hardly shabby magnets of that strength are used in MRI machines it's still pretty limiting for many scientific and other applications. Theoretically you could make more powerful permanent magnets, but with all that metal in it, it would be extraordinarily heavy and impractical.
Luckily, there's more than one way to make a magnet. Take electromagnets: They aren't permanent, but they can be far stronger than the strongest permanent magnet.
But before we get to the really heavy stuff, let's start with other, smaller types of temporary magnets.
Click here to open a new window and see a random smattering of soft iron filings. (Iron nails or paper clips would also do the trick here).
Nothing special about these filings. Pretty hum-drum.
Electric motors are made with magnets.
Now click the "on" button to expose these filings to a magnetic field and see what happens.
Presto-chango! Now that they're in a magnetic field, these filings have themselves become magnets. Just like the piece of iron you saw in the previous section. Except that these filings won't retain the property in the absence of a field. The second you take the magnet away, they're demoted again from miniscule magnets to run-of-the-mill iron filings. Easy come, easy go. Soft iron and other types of iron alloys behave this way. That's why they are used as temporary magnets in telephones and electric motors.
Let's move on to a type of temporary magnet that can flex some more muscle.
Electromagnets: Two Forces Team Up
Resistive electromagnets, another type of temporary magnet, combine the powers of electricity and of magnetism to enormous effect. Although superconducting electromagnets, which we'll discuss soon, offer many advantages, certain laws of physics prevent them from reaching the field strengths electromagnets are capable of.
Some of the world's most powerful resistive magnets here at the MagLab; they can generate a sustained magnetic field of 35 tesla. (We're not counting here our world-record hybrid magnet or the stronger, short-lived fields of pulsed magnets, both of which we'll explain shortly.) Scientists use these high fields to do a wide range of experiments concerning physical, chemical and biological processes of different types of matter.
But electromagnets don't have to be hugely powerful. Whether in your toaster or TV, even small ones can be quite useful. To make one, you start out with some wire.

Now, plug that wire in so that a current runs through it. The result? A magnetic field (depicted in blue) – and a temporary magnet that you switched on with the current. Think of this as the simplest kind of electromagnet.

Turns out, there's a magnetic field circling every electrical wire, a fact famously stumbled upon by an astounded Dane named Hans Christian Ørsted in 1819, who noticed the magnetic needle of a compass align itself perpendicularly to a nearby current-carrying wire.
Physics Factoid
Ever hear of "animal magnetism"? Some doctors once believed magnetic fields ran through the human body, and that applying magnets to them could cure various ills. Some people today believe magnets have special healing properties.
That's because electrical current – the flow of electrons through a wire – always generates a magnetic field. You can tell which way the invisible lines of that field are traveling: If you point the thumb of your right hand in the direction of the current, the magnetic field travels in the direction that your fingers, curved as if clasping a rod, are pointing. (This web site features an interactive tutorial on the magnetic field around a wire, if you'd like to learn more.)
The magnetic field around a household electrical wire, and household appliances, is pretty small. About a foot away from a whirring ceiling fan, for example, the magnetic field measures just a few milligauss. To create a much more powerful magnet, we need to take it to the next level. Let's see what happens when we take that same wire and coil it around once in a circle.

What do you know? With that simple step, all the smaller, separate magnetic fields circling the wire have joined forces to create a far, far greater magnetic field.
If that's the effect of creating a single coil, what will happen if we make a bunch of them 10, let's say? Let's try it and find out.
Solenoid
Very cool! Our new magnetic field is about 10 times more powerful. This special type of coil, by the way, is called a solenoid, and the magnetic field it produces increases proportionately to the number of coils you include. Coiling the wire also makes the field more uniform, a property important to scientists testing the effects of magnetic fields on different materials.
We can improve the magnetic field power of this solenoid even more by inserting an iron alloy core in the middle. Remember our iron atoms of earlier, and how each was a tiny little magnet? Well, when you put the iron alloy core into a magnetic field, all the atoms in the iron align with it and, in so doing, boost the magnet's field strength significantly yet without using more electricity! Speaking of electricity, the more power you add to your solenoid, the greater your magnetic field.
Bitter plate
You can improve on this basic solenoid by replacing the coils with specially designed Bitter plates that better withstand both the pressure resulting from high magnetic fields and the heat resulting from electrical current. These plates were first invented by a fellow named Francis Bitter in 1936. In the 1990s MagLab scientists greatly improved on his design, inventing the Florida Bitter plate, which enabled the creation of more powerful magnets. Most large electromagnets used for research use Bitter plates, which is why they are sometimes called "Bitter magnets."
These electromagnets are called "resistive" magnets because, as in any machine running on normal electricity, the electrons that make up the current encounter resistance as they bump into atoms and other electrons along their journey. This inefficiency costs when it comes time to pay the electric bill; in fact, the Magnet Lab uses about 7 percent of the electricity consumed in Tallahassee, a city of about 160,000 residents! If we didn't have magnets to operate, our on-site power source could light up some half-million 100-watt light bulbs at the same time! The MagLab also uses a considerable amount of water, which is forced through the holes of the Bitter plates to prevent the magnets from getting too hot.
So resistive magnets eat lots of electricity and drink lots of water, two expensive habits. This is where superconducting magnets, the next type of temporary magnet we will explore, offer some advantages.
Superconducting Magnets: Power Unplugged
Superconductivity is a very cool phenomenon in which electrical resistance is overcome with the help of very cold temperatures colder than anything this side of Pluto!
Physics Factoid
Most superconducting magnets are made using an alloy called niobium-titanium "Ni-Ti" for short.
You could think of it this way: In a superconducting current, the atoms that make up the conducting material stay the heck out of the way of the electrons that make up the current: They're just too cold to make any trouble! It's easy street for those electrons; once you get 'em started, they'll chug along well after you've unplugged the thing, as long as you keep things chilly.
Superconducting magnets are powered this way, rather than by conventional electricity. While you save on the electric bill, these magnets are more expensive to build, and you need a constant supply of cryogens liquid helium and liquid nitrogen to keep them good and cold. In fact, if you look at a superconducting magnet (such as the one pictured below), you'll notice that most of it is made up of the materials devoted to keeping it cold.

Superconductivity is an awesomely powerful phenomenon, one that scientists have only just begun to exploit. For years doctors have used it in the powerful superconducting magnets of MRI machines, which take noninvasive pictures of their patients' insides. Researchers are also aggressively studying how superconducting magnets can be used in levitating trains.
The Magnet Lab is home to one of the most powerful superconducting magnets on the planet, a 900-megahertz machine used for nuclear magnetic resonance (NMR) studies that delivers a field of 21 tesla. At Japan's National Institute for Materials Science, a slightly more powerful superconducting magnet is in operation. Magnet designers continue to push the envelope, struggling to overcome problems with critical fields, the point at which superconducting materials cease to superconduct. At the Magnet Lab, scientists are working on superconducting magnets with fields upwards of 38 tesla; they have already demonstrated the technology to make these magnets.

The 900 MHz Ultra-Wide Bore Magnet
Let's see. We've learned that the highest fixed field created by a superconducting magnet is about 21.6 tesla, and the highest field created by a resistive electromagnet is 35 tesla.
So you can't get any higher with a steady magnetic field?
Sure you can. You just need to do a little math!
Hybrid Magnets: Best of Both Worlds
Unlike most of the math that goes on at the Magnet Lab, the equation you need to make a more powerful continuous field magnet is pretty easy. Take a very powerful superconducting magnet say, 11.5 tesla and a very powerful resistive magnet say, 33.5 tesla and put them together. What do you get?
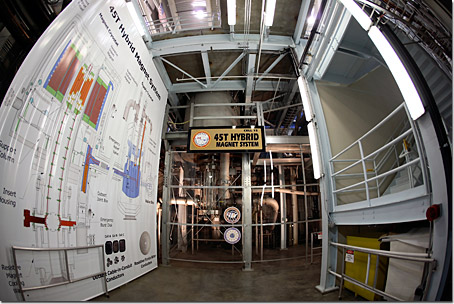
You get a magnet mutt (although around here we prefer the term hybrid). The combo produces a magnet with the most powerful sustained field in the world: the 45 tesla hybrid magnet designed and operated here at the lab. This 35-ton behemoth is in wide demand among scientists across the globe.
Physics Factoid
There are almost 4 miles of superconducting cable in the Magnet Lab's 45 tesla hybrid magnet.
Though designs of hybrid magnets differ, the MagLab hybrid consists of a resistive magnet encircled by a superconducting magnet. Most of the 22-foot tall device is made up of systems that keep the magnet cold enough to operate. Vast amounts of deionized water are used to keep the Bitter magnet cool – about 400 liters a second.
One of only a handful of hybrids in the world, this mighty marvel uses in its superconducting portion enough copper wiring for 80 average homes. And that part of the magnet is kept mighty cold (in the neighborhood of -452 degrees Fahrenheit, or -269 degrees Celsius) almost all the time, even when not in use. If the magnet gets to room temperature, it takes at least six weeks to cool it down to operating temperature!

As impressive as this tool is, magnet engineers here at the lab, widely recognized as the best in the world at what they do, are already at work creating the next generation of hybrid. The Series Connected Hybrid will generate high magnetic fields while using just one-third the power of traditional "all-resistive" magnets.
Pulsed Magnets: Brief Shining Moments
So, 45 tesla is the greatest field strength ever achieved by a magnet?
Yes and no. It's the greatest continuous field strength ever achieved by a magnet. But a type of magnet called a pulsed magnet can achieve far higher strengths. But there's a trade-off: The field lasts just a few seconds or less.
The Magnet Lab has several of these magnets, which were designed and built in Tallahassee then shipped to the lab's Pulsed Field Facility at Los Alamos National Laboratory for operation. Designers must choose their materials carefully, taking into account the incredible stresses and heat produced by such enormous magnetic fields.
Pulsed magnets come in two types, destructive and non-destructive, the latter of which we'll discuss first.
Non-destructive magnets can be short-pulse, long-pulse. The "short" vs. "long" is a reference to how long the magnetic field lasts. In this context, long is relative at most 2 seconds. Short is measured in milliseconds. Long-pulse magnets are motor-generator driven, and short-pulse are fueled by capacitors. In either case, they work pretty much the same way.
Physics Factoid
The incredible field strengths achieved by these destructive pulsed magnets are over before the operator's finger leaves the "On" button less than 10 microseconds. A microsecond is 0.000001 second.
The magnets are impressive for the fields they generate and the amount of energy they consume doing it. The Magnet Lab's most powerful non-destructive magnet generates a field of 100 tesla. When turned on, this multi-shot magnet contains the energy of more than 100 sticks of dynamite and is the most powerful magnet of its type in the world. Magnet designers are further developing that magnet, which came online in October 2006, in the hope that it will eventually reach 100 tesla. The project is funded by the U.S. Department of Energy and the National Science Foundation.
If you're ever in the vicinity when one of these magnets is in operation, be sure to use earplugs. It emits a kind of shriek that has been compared to Godzilla on a rampage. That's because these tremendous magnetic forces produce enormous pressure on the order of 200,000 pounds per square inch. That's one reason the magnet operates so briefly: Left to run any longer, it would either succumb to pressure or melt from the awesome heat generated by all the power surging in.
An exploding pulsed magnet.
While scientists usually try to avoid destroying their equipment, they are sometimes willing to do just that, in exchange for a phenomenal magnetic field. In fact, in destructive magnets, scientists actually detonate explosives around the pulsed magnet at the same time they turn it on. This compresses and boosts the magnetic field to as high as 1,000 tesla for the few microseconds before the whole shebang explodes. Now that's what you call a temporary magnet!
You may well wonder what the point of such an exercise is: After all, you're not just blowing up a magnet, you're blowing up your whole experiment! Truth is, some destructive magnets (called single-turn magnets) don't really blow up they blow outwards so the sample is conserved. For those magnets that do blow up, scientists have become adept at measuring and collecting data during their microseconds-long window of opportunity so all is not lost, after all!
So there you have it: From your fridge to your hospital's MRI clinic, from an infinitesimal electron to the MagLab's 35-ton hybrid. Magnets and their fields are everywhere, hard at work in innumerable ways and forms. That's our story and we're sticking to it!
Thanks to this story's science adviser, Deputy Lab Director Eric Palm.
By Kristen Coyne