At the National High Magnetic Field Laboratory, we've teamed up science and athletics to invent a few new sports inspired by the cool things scientists study in high-field magnets.
Some of the things scientists study are spherical, like the golf, tennis and soccer balls we all know so well. It turns out that where there's a will — and a sphere and a little imagination — there's a new sport waiting to be thought up.
Our imagined pastimes probably won't replace the World Series or Olympics, but they do offer a glimpse into the amazing things scientists discover using high-field magnets. They might even inspire you to invent and play a science sport of your own.
So let's get this SciBall rolling!
Ultimate Thermoelectrics
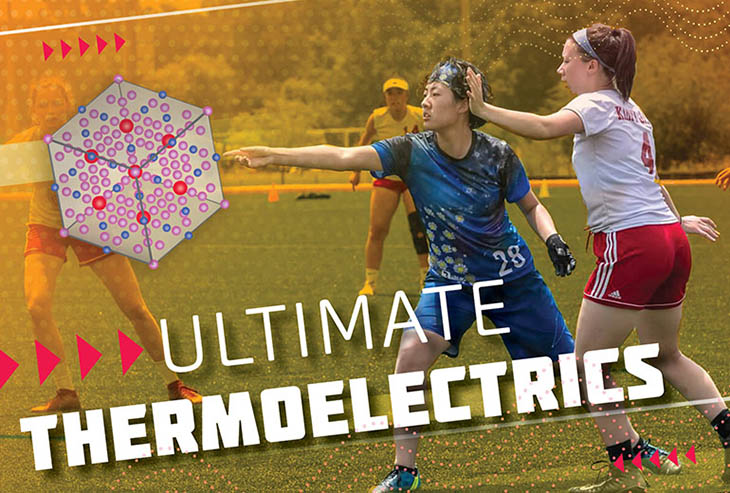
Photo illustration by Caroline McNiel, based on a photo by Savage Frieze and an illustration of the crystal structure of a “1-2-20” thermoelectric material made of ytterbium, zinc and lanthanum.
Thermoelectric materials can conduct electricity if there is a temperature difference between the two ends (or conduct heating and cooling if there is a voltage difference). It's a nifty trick that few materials can pull off because the movement of electrons and of heat usually go hand in hand. But to have good thermoelectric performance, these two properties need to be decoupled. You want to encourage the movement of electrons for the current, while at the same time discouraging phonons from transporting heat. The heat gradient needs to be preserved to maintain the flow of electricity.
It's a challenging puzzle — sort of like figuring out how to transfer an object from one end of a playing field to the other without moving your feet. In the sport of Ultimate, players overcome that constraint by tossing the disc from teammate to teammate until, perhaps in a dramatic play called a layout, someone catches it in the end zone. Thermoelectrics are important because they can be used for everything from power generation in space to devices that recoup energy wasted in cars through heat.
At the National MagLab, scientists use high-field magnets to study compounds with these properties, including 1-2-20s, a promising class of materials with a round, cage-like structure that sidelines phonons while letting electrons fly through like a well-hucked disc.
MOFfleball
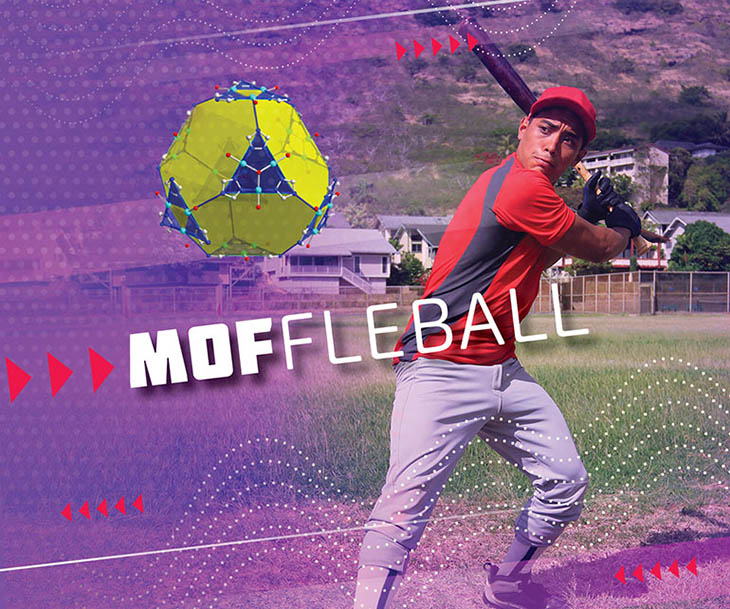
Photo illustration by Caroline McNiel, based on a photo by Michael Mitchell. MOF illustration, based on a 1,2,4-triazolyl-carboxylate-based MOF, adapted with permission from Inorg. Chem. 2014, 53, 7, 3642–3654, copyright 2014 American Chemical Society.
You know how waffles have all those little nooks and crannies to soak up the maple syrup? So do special molecules known as metal organic frameworks — or MOFs (rhymes with moths), as the scientists who make and study these lattice structures call them.
Researchers are interested in MOFs in part because you can cram an astonishing amount of stuff inside them. In the sports world, an apt MOF analogy would be a hole-pocked, stuffable wiffleball. MOFs are designed to be quite porous and contain a remarkable amount of surface area. If you were to take all the surfaces hiding inside these tiny structures and lay them out flat, they could easily cover, say, an entire wiffleball field. This makes them great at absorbing, storing and transporting other compounds.
MOFs could be used in storing energy, gas and liquids, purifying water, catalysis, drug delivery, even carbon sequestration — a veritable science grand slam. It’s easy to see why MOF researchers come to the MagLab, the big league for strong and unique magnets. The lab is home base to the world’s most powerful nuclear magnetic resonance magnet, the 36-tesla Series Connected Hybrid, the sweet spot for analyzing complex structures like MOFs. With that kind of high-field advantage, scientists can’t help but hit it outta the park.
Fermi Football
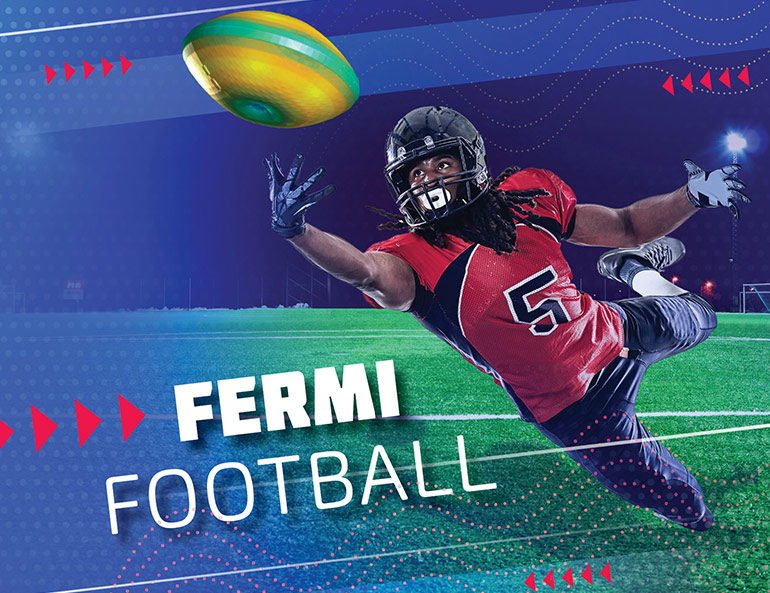
Photo illustration by Caroline McNiel, based on a photo by Franck Camhi. Illustration of a Fermi surface courtesy of Amalia Coldea, adapted after work performed in Phys. Rev. Research 2, 012055 (2020).
Many physicists are obsessed with a kind of electron they call Fermi electrons. In the systems they study, these are the MVP electrons — the ones that do interesting things, especially when exposed to high fields. While other electrons are sitting on the sidelines because they’re too tightly bound to a nucleus or too high in energy to interact in any relevant way, Fermi electrons are situated on what physicists call the Fermi surface. Think of the Fermi surface as the part of the field in American football where all the action is going on — the line of scrimmage.
Physicists can observe these electrons by putting the material they are studying in a very high magnetic field, running a current through it and measuring its resistance. They are looking for quantum oscillations — discrete jumps in the resistance that indicate that those Fermi electrons have jumped up from one quantum energy level to the next. Imagine the Fermi electrons as little quantum quarterbacks who need to chuck the pigskin one discrete unit downfield — the quantum equivalent of 10 yards — to earn a first down. The crowd goes wild, and physicists record a quantum oscillation.
Through some tricky math, physicists can then take their measurements and create a ball-like representation of it called a Fermi surface. It's a kind of topological map that tells scientists tons about these Fermi electrons' behavior and about the properties of the material they inhabit. For high-field physicists, it's an insight equivalent to a game-winning touchdown.
Virus Volley
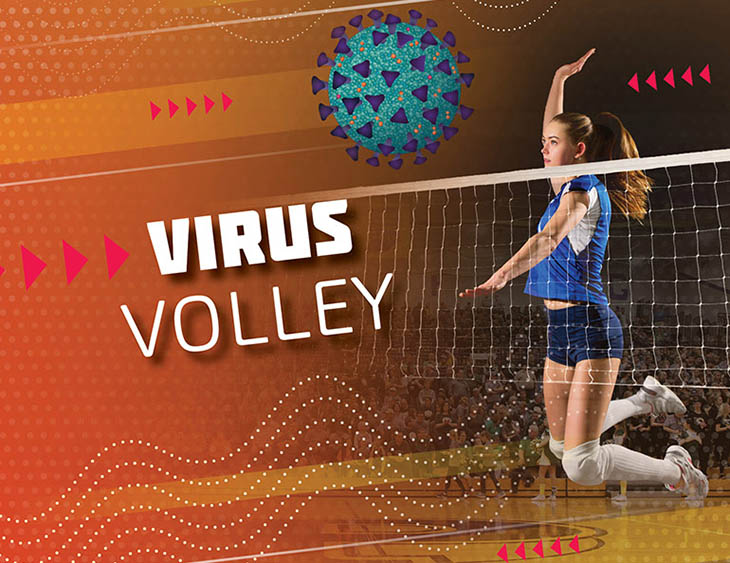
Photo illustration by Caroline McNiel, based on a photo by Boris Ryaposov.
One ball we've seen far too much lately is the SARS-CoV-2 virus, which causes COVID-19. Scientists around the globe, including at the National MagLab, have been working hard to snuff out the hated orb. Volleyball seems the most apt sports metaphor: Picture a player leaping at the net, arm stretched high, poised to spike (or kill, in the parlance of the sport) the spikey sphere.
At the National MagLab, scientists are using nuclear magnetic resonance (NMR) and magnetic resonance imaging (MRI) to study the virus in the hopes of contributing to its undoing. The lab's powerful magnets help them understand the structure and behavior of the constituent proteins, looking for a hole in the viral membrane's defense that a drug could attack. MagLab scientists and engineers are also developing COVID-crushing stem cell therapies that they plan to test in animal models using the world's strongest MRI machine for animal research. Together, these researchers form an imposing front row working to block the virus.
Hydride Hockey

Photo illustration by Caroline McNiel, based on a photo by Dusan Petkovic. Illustration of lanthanum hydride molecule by Kevin John.
A superhydride is a molecule made up of a bunch of hydrogen atoms that form a cage, imprisoning one or more other atoms inside, like a sidelined hockey player waiting it out in the penalty box. When placed under enormous pressure, superhydrides have been shown to be superconducting at record-warm temperatures. Superconductors, unlike conventional conductors such as copper, can transport electricity with perfect efficiency because electrons encounter no friction whatsoever. You might say it's the difference between laboriously trudging through snow and skating effortlessly across a smooth sheet of ice.
Until relatively recently, all known superconductors worked only at cryogenic temperatures many times colder than a hockey rink. But superhydrides have dashed toward the milestone of room temperature with the blistering speed of a slap shot. Using the strongest continuous-field magnet in the world, physicists have been probing these extraordinary compounds and testing different recipes, hoping to find the right combination to finally cross the goal line they've been skating around for years.
Bucky Ball
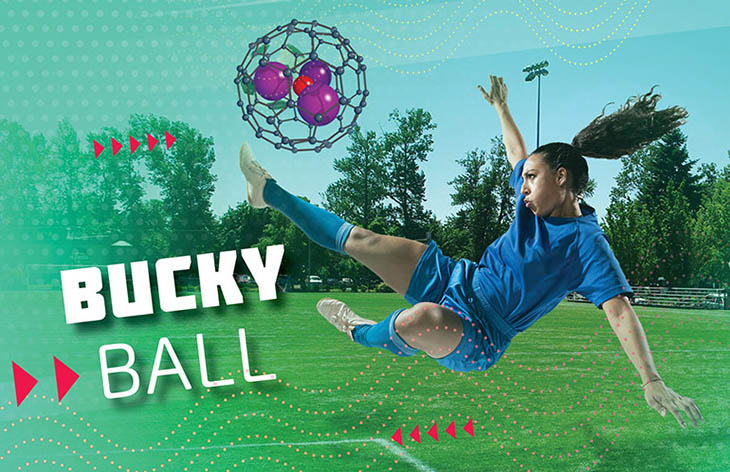
Photo illustration by Caroline McNiel, based on a photo by FREEPIK2 and an illustration of a carbon nanocage.
In 1985, scientists zapped a pile of graphite with a laser and discovered a strange new molecule. Made of 60 carbon atoms, it resembled the white and black sphere known as a football in most of the world and a soccer ball in North America.
Its discoverers dubbed it a buckminsterfullerene (buckyball for short) after an architect who designed geodesic domes similar in shape. One of the many discoveries to come from that Nobel Prize-winning feat are carbon nanocages, molecules made of 80 carbons that encapsulate other atoms.
Scientists have kicked around ideas for different nanocage recipes. They vaporize varying mixes of graphite and other elements, then study the results in an ion cyclotron resonance mass spectrometer to better understand what they have created. Each variation has its own structure, properties and potential game-changing uses in health and energy. Some nanocages, for example, could transport tiny cargoes inside the human body, such as drugs or MRI contrast agents.
Now that's what we call using your head!
How to become a SciBall Superstar
Love these science sports? Want to make your SciBall trading card? Here's the play by play (a basic familiarity with PowerPoint, Google Slides or Keynote is needed).
- Step 1: Download one of the trading cards below.
- Step 2: Take a picture of yourself playing the sport. (Think catching, kicking, throwing or spiking or batting and invisible ball.)
- Step 3: Click on the name of the sport and temporarily drag that image layer to the side.
- Step 4: Right click on "Your Photo Here" and select “Change Picture” to insert your photo. Reposition or zoom in as needed. Reposition the image layer you dragged off to the side back into place.
- Step 5: If you need to reposition the SciBall, just click on it to drag it around to the perfect spot to complete your image.
- Step 6: Fill in your name, nickname and the team you play for. Get creative, nerdy, and silly.
- Step 7: Click on “File” then on “Export” to save your file as a jpg or other file format.
- Step 8: "Trade" your SciBall card with your friends on social media. Don't forget to tag @NationalMaglab and #SciBall.
- Step 9: Choose another SciBall sport and make another card! Collect 'em! Trade 'em! Post 'em!
Thanks to MagLab physicist and DC Field Facility Director Tim Murphy; MagLab chemist Andrzej Ozarowski; and MagLab physicist Kaya Wei, who served as science advisors for parts of this story.
Story by Public Affairs Team